Our understanding of nucleosynthesis in the Universe is mainly due to the strong interaction between astronomical observations, nuclear physics studies and astrophysical modeling. These fields are in constant development: new telescopes and satellites open more and more windows on the Cosmos, nuclear physics measurements are pushed to the limits thanks to new facilities (radioactive beams, high-intensity stable beams) and sophisticated detection systems, and nuclear physics calculations benefit from state-of-the-art computing methods and power.
Thermonuclear reaction rates and β-decay rates are key ingredients needed to understand the production of elements in the Universe. Their determination requires many nuclear physics inputs: the reaction cross sections, and the mass, β-decay, and spectroscopic properties of the involved nuclei. However the associated experimental studies remain very challenging either because of the very weak cross sections or because of the radioactive nature of the involved nuclei. On the theoretical side, the global testing and comparison of nuclear models providing information over the nuclear chart is still in order.
Gamma-ray astronomy in the MeV region plays a special role in the observational study of nucleosynthesis because it enables direct probing of the nuclear processes at work. The INTEGRAL and RHESSI spacecraft telescopes are near the end of their life, and a nationwide investigation of the best new generation of satellites is under way.
The study of nucleosynthesis at IJCLab follows mainly three investigation lines: the experimental determination of relevant reaction rates (mainly indirect cross-section measurements and β-decay studies) in several astrophysical environments, theoretical calculations, and the development of an instrument observing the sky in the MeV range.
Explosive nucleosynthesis in classical novae and Type I X-ray bursts
Classical novae and Type I X-ray bursts are explosive events that take place in close binary systems consisting of a white dwarf (for novae) or a neutron star (for X-ray burst) accreting rich H and He material from a large main sequence (or a more evolved) star. Our pole is involved in improving the knowledge of cross sections of key nuclear reactions involved in these phenomena, which is crucial for the interpretation of observations and the validation of the underlying astrophysical models describing these astrophysical sites. Experiments are performed at ALTO with the Split-Pole spectrometer or at GANIL.
Quiescent and explosive nucleosynthesis in massive stars
Massive stars play an essential role in the chemical evolution of the galaxy and are responsible for the synthesis of “most” of the elements present in the universe. These are produced either during their quiescent burning phases or during their explosive phase. Among the nucleosynthesis products of these stars, several are gamma emitters such as 26Al, 44Ti and 60Fe, making them potential targets for space gamma-astronomy missions; and others are observed in presolar grains such as 13C, 14N, 15N. We studied various key reactions involved in massive stars these last five years via transfer or inelastic scattering reactions using the Split-Pole and Q3D magnetic spectrometers at the ALTO and MLL-Munich facilities.
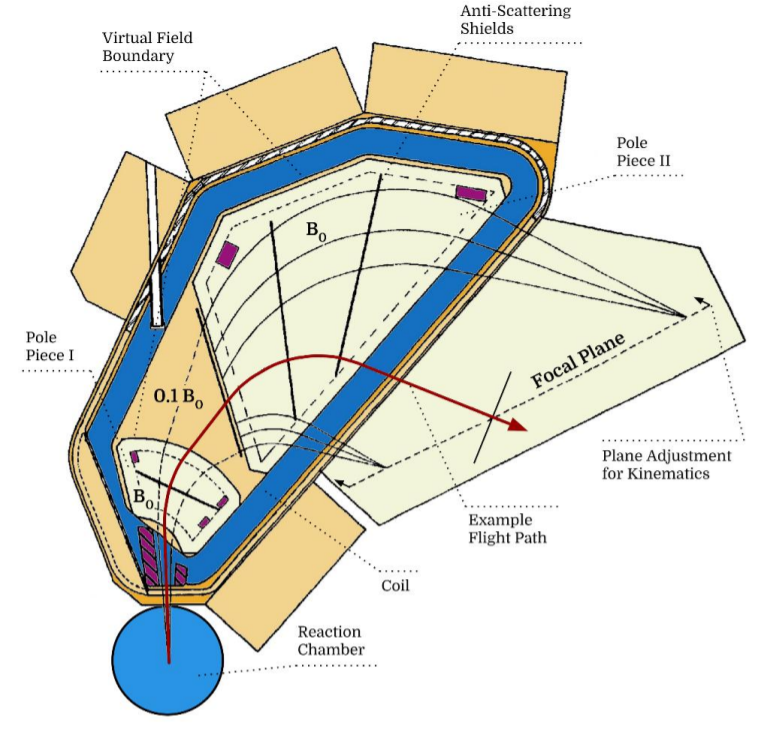
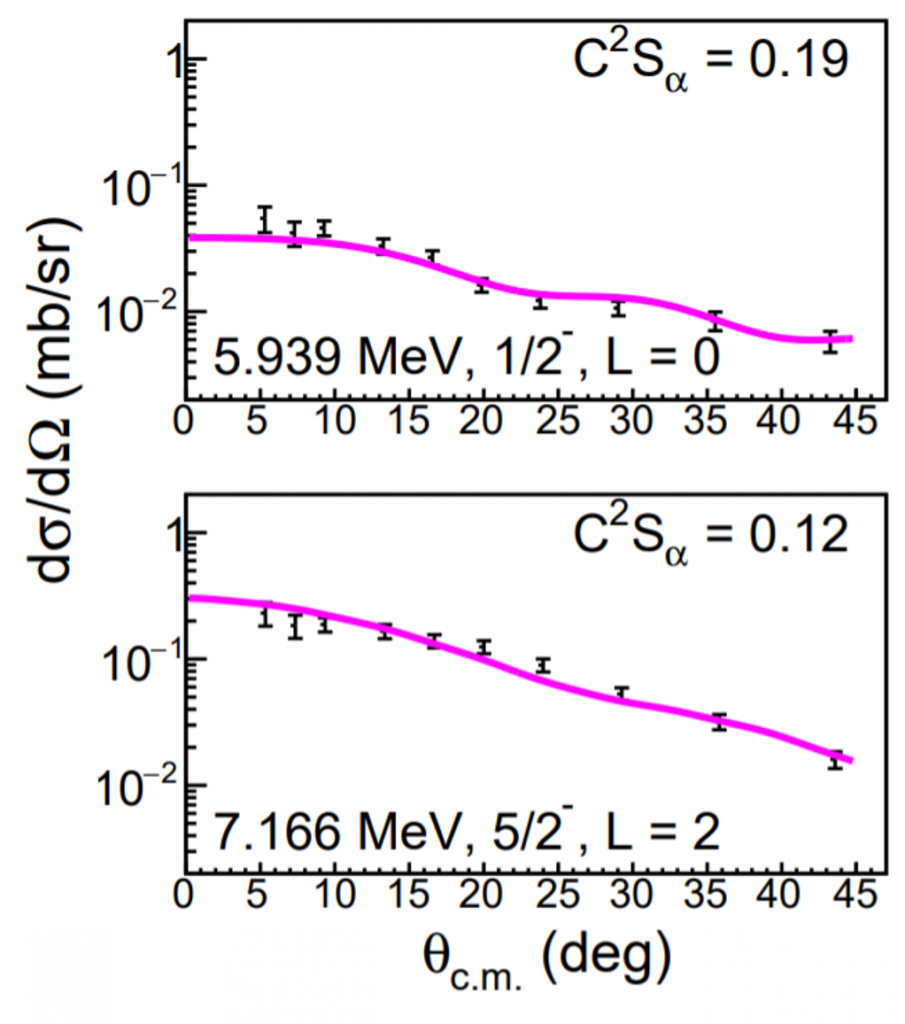
Drawing of the Orsay Split Pole and recent experimental results showing the differential cross-section of the 13C(7Li, t)17O reaction.
Abundance anomalies in globular clusters
Globular clusters are vital testing grounds for models of stellar evolution and the early stages of the formation of galaxies. Abundance anomalies in some globular clusters, such as the enhancement of potassium and depletion of magnesium, can be explained in terms of an earlier generation of stars polluting the presently observed stars. The potential range of temperatures and densities of the polluting sites depends on the strength of a number of critical reaction rates. A study of relevant reactions is performed with the Q3D magnetic spectrometer at MLL-Munich and with the DRAGON recoil spectrometer at TRIUMF.
The r-process
About half of the abundance of the elements beyond iron are produced by the r-process which is a succession of rapid radiative neutron captures followed by β-decays. The r-process path extends far from the valley of stability towards the neutron dripline. r-elements solar abundance exhibit strong peaks that are correlated with the neutron shell closures indicating that the nuclear structure plays an important role.
The knowledge of nuclear input parameters, such as nuclear masses, beta-decay half-lives, beta-delayed neutron emission probabilities and neutron-capture rates, are essential for modeling the r-process. The nuclear pole is involved in the determination of these parameters by measurements at different radioactive ion-beam facilities: beta-decay measurements to populate pygmy dipole resonances at ALTO, as well as mass measurements and decay spectroscopy at ISOLDE and Jyväskylä.
Toward a spatial spectro imager in the MeV range
There is a general agreement in the community that the next γ-ray observatory operating in the MeV range will be a Compton telescope. One possible implementation of such concept consists in the use of a silicon tracker to record the Compton interactions and the pair production by gamma rays above a few MeV. The energy of the scattered photons or e–/e+ pairs is measured in a calorimeter made of scintillators. The development of a small prototype of a γ-ray Compton spectro-imager is undertaken in strong collaboration with the A2C pole since several years through the ComptonCAM project. The nuclear pole is in charge of building and characterizing the tracker based on double sided silicon stripped detectors (DSSSD). Dedicated boards have been designed for hosting the detectors together with the front-end and back-end electronics. Performances achieved in term of spectral resolution and energy threshold correspond to the specifications.